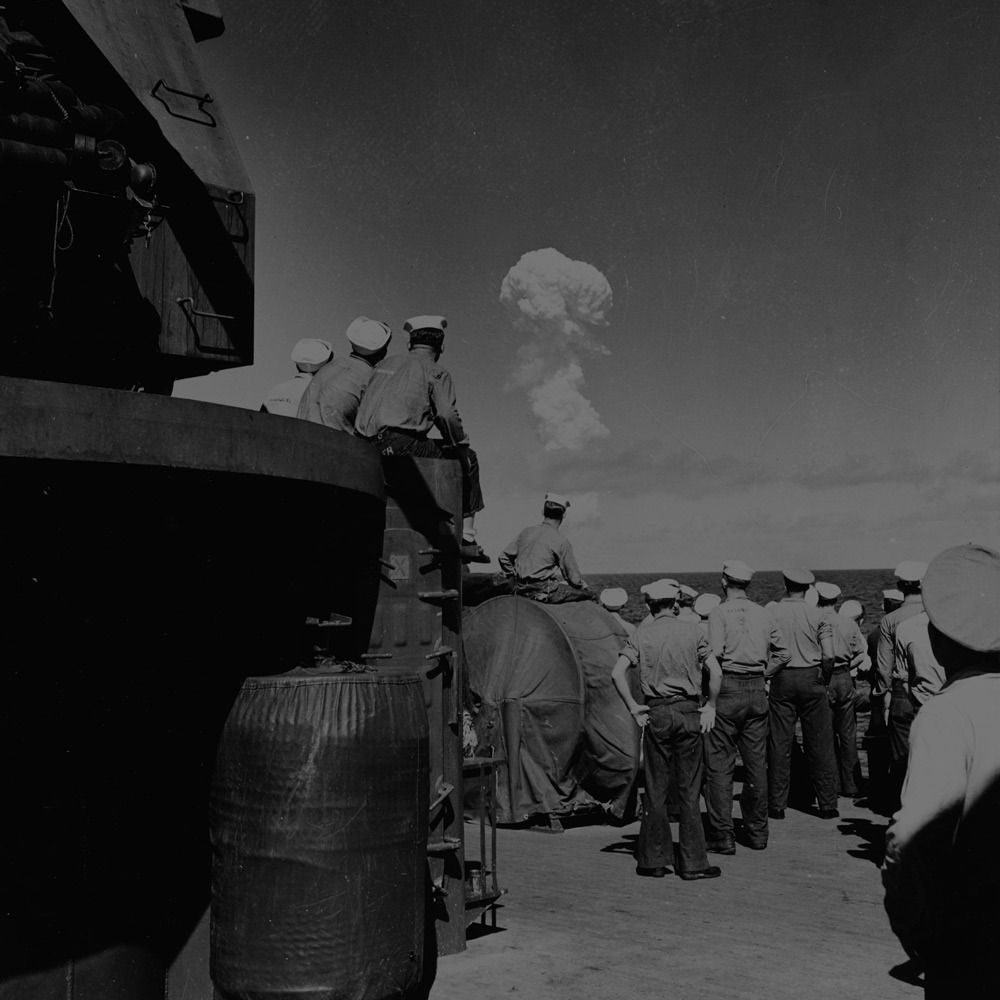
URANIUM
THE PROJECT IN ACTION
​
​
The key raw material for the project was uranium, which was used as fuel for the reactors, feed that was transformed into plutonium, and in its enriched form, in the atomic bomb itself. There were four known major deposits of uranium in 1940: in Colorado, in Northern Canada, in Joachimsthal, Czechoslovakia, and in the Belgian Congo. Nichols arranged with the State Department for export controls to be placed on uranium oxide and negotiated for the purchase of 1.1 Million kg of uranium ore from the Belgian Congo and the remaining stocks of mined ore stored in the Congo. He also negotiated with Eldorado Gold Mines for the purchase of the desired ore from its refinery in Port Hope, Ontario.
The American and British leaders concluded that it was in their countries' interest to gain control of as much of the world's uranium deposits as possible. The richest source of ore was the Shinkolobwe mine in the Belgian Congo, but it was flooded and closed. Nichols unsuccessfully attempted to negotiate the reopening and sale of the entire future output to the United States with Edgar Sengier, the director of the company that owned the mine, the Union Minière du Haut-Katanga. Sir John Anderson and Ambassador John Winant hammered out a deal with Sengier and the Belgian government in May of 1944 for the mine to be reopened and 1.6 Million kg of the ore to be purchased at $2.9 for a kilogram. Groves also arranged for the purchase of US Vanadium Corporation's stockpile in Uravan, Colorado. Mallinckrodt Incorporated in St. Louis, Missouri, took the raw ore and dissolved it in nitric acid to produce uranyl nitrate. Ether was then added in a liquid–liquid extraction process to separate the impurities from the uranyl nitrate. By July of 1942, Mallinckrodt was producing almost 1,000 kg of pure oxide a day, but turning this into uranium metal initially proved more difficult for contractors.
​
​
​
​
​
​
​
​
​
​
​
​
​
​
​
​
​
​
​
Only 0.7% of the naturally occurring uranium, which is 99.3% uranium-238, is actually fissile. The more common isotope must be physically separated from the chemically identical uranium-235. There were several options for uranium enrichment, most of which took place at Oak Ridge.
The centrifuge, which was the most obvious technology, failed, but other successful technologies included electromagnetic separation, gaseous diffusion, and thermal diffusion. Groves came up with the concept of using the output of some factories as the input for others in February of 1943.
​
​
​
​
​
​
​
​
​
​
​
​
​
​
​
​
​
​
​
​
​
​
​
​
​
​
​
​
​
​
​
​
​
​
​
​
The centrifuge process was regarded as the only promising separation method in April 1942. Jesse Beams had developed the process at the University of Virginia during the 1930s, but encountered many technical difficulties. He began working with uranium hexafluoride and was able to separate uranium-235. Karl P. Cohen investigated the process and produced a body of mathematical theory making it possible to design a centrifugal separation unit. However, frequent failures of motors, shafts and bearings at high speeds delayed work on the pilot plant.
In November of 1942, the centrifuge process was abandoned by the Military Policy Committee, but research into it advanced significantly after the war with the introduction of the Zippe-type centrifuge. It eventually became the preferred method of Uranium isotope separation, being far more economical than the other separation methods used during World War II.
​
​
​
​
​
​
​
​
​
​
​
​
​
​
​
​
​
​
​
​
​
​
​
​
Electromagnetic isotope separation was developed by Lawrence at the University of California Radiation Laboratory and employed devices known as calutrons, a hybrid of the standard laboratory mass spectrometer and the cyclotron magnet. The process was neither scientifically elegant nor industrially efficient, but was approved because it was based on proven technology and represented much less risk. Marshall and Nichols discovered that the electromagnetic isotope separation process would require 4.5 million kg of copper, but silver could be substituted in an 11:10 ratio. Silver bars were cast into cylindrical billets and taken to Phelps Dodge in Bayway, New Jersey, where they were extruded into strips 15.9 mm thick, 76 mm wide and 12 m long. After the war, all the machinery was dismantled and cleaned and the floorboards beneath the machinery were ripped up and burned to recover minute amounts of silver.
​
Construction of the electromagnetic separation plant, known as Y-12, was assigned to Stone & Webster by the S-1 Committee in June 1942. The design called for five first-stage processing units, known as Alpha racetracks, and two units for final processing, known as Beta racetracks. The Y-12 plant was started up for testing in October of 1944, but the 12,700 kg vacuum tanks crept out of alignment due to the power of the magnets used and as a result the magnetic coils started shorting out. In December, Groves ordered a magnet to be broken open and handfuls of rust were found inside. The magnets were then sent back to the factory to be cleaned. The second Alpha I was not operational until the end of January of 1944, the first Beta and first and third Alpha I's came online in March, and the fourth Alpha I was operational by April. The four Alpha II racetracks were completed between July and October of 1944.
​
Tennessee Eastman was contracted to manage Y-12 on the usual cost plus fixed-fee basis, with a fee of $22,500 per month plus $7,500 per racetrack for the first seven racetracks and $4,000 per additional racetrack. The calutrons were initially operated by scientists from Berkeley to remove bugs and achieve a reasonable operating rate, but were then turned over to trained Tennessee Eastman operators who had only a high school education. Nichols compared unit production data, and pointed out to Lawrence that the young "hillbilly" girl operators, known as Calutron Girls, were outperforming his.
​
​
​
​
​
​
​
​
​
​
​
​
​
​
​
​
​
​
​
​
The most promising but also the most challenging method of isotope separation was gaseous diffusion. Graham's law states that the rate of effusion of a gas is inversely proportional to its molecular mass, so in a box containing a semi-permeable membrane and a mixture of two gases, the lighter molecules will pass out of the container more rapidly than the heavier molecules. Research into the process was carried out at Columbia University by Harold Urey, Karl P. Cohen, and John R. Dunning. In November 1942, M. W. Kellogg accepted an offer to construct the plant, codenamed K-25.
​
The process faced formidable technical difficulties, such as the highly corrosive gas uranium hexafluoride, the motors and pumps having to be vacuum tight and enclosed in inert gas, and the design of the barrier, which had to be strong, porous and resistant to corrosion by uranium hexafluoride. Edward Adler and Edward Norris created a mesh barrier from electroplated nickel, but the Norris-Adler prototype proved to be too brittle. Kellex's design for K-25 was a four-story 0.8 km long U-shaped structure containing 54 contiguous buildings divided into nine sections. Work on the main building began in October of 1943, and the six-stage pilot plant was ready for operation by the 17th of April, 1944. Groves cancelled the upper stages of the plant, directing Kellex to instead design and build a 540-stage side feed unit, which became known as K-27. Kellex transferred the last unit to the operating contractor, Union Carbide and Carbon, on the 11th of September, 1945.
​
The production plant commenced operation in February of 1945, and the quality of the product increased. By April of 1945, K-25 had attained a 1.1% enrichment and the output of the S-50 thermal diffusion plant began being used as feed. K-25 and K-27 achieved their full potential only in early post-war period, when they eclipsed the other production plants and became the prototypes for a new generation of plants.
​
​
​
​
​
​
​
​
​
​
​
​
​
​
​
​
​
​
​
​
​
​
The thermal diffusion process was based on Sydney Chapman and David Enskog's theory that when a mixed gas passes through a temperature gradient, the heavier one tends to concentrate at the cold end and the lighter one at the warm end. This process was developed by US Navy scientists, but was not one of the enrichment technologies initially selected for use in the Manhattan Project due to doubts about its technical feasibility. In April of 1944, Captain William S. Parsons, the naval officer in charge of ordnance development at Los Alamos, brought Oppenheimer the news of encouraging progress in the Navy's experiments on thermal diffusion. Groves set up a committee to investigate the idea and estimated that a thermal diffusion plant costing $3.5 million could enrich 50 kilograms of uranium per week to nearly 0.9% uranium-235. Groves approved its construction on the 24th of June, 1944, and contracted with the H. K. Ferguson Company of Cleveland, Ohio to build the plant. The S-50 plant was built to produce uranium-235 at a pressure of 690 kPa and a temperature of 285 °C. Work commenced on the 9th of July, 1944, and the plant began partial operation in September of the same year. In June of 1945, the plant produced 5,770 kg of uranium-235. In March 1945, all 21 production racks were operating and the uranium was fed into Y-12. In July of 1945, 50 kilograms of uranium enriched to 89% uranium-235 was delivered to Los Alamos. The entire 50 kg, along with some 50%-enriched, were used in the first atomic bomb; Little Boy.
​
​
​
​
​
​
​
​
​
​
​
​
​
​
​
​
​
​
​
​
​
​
​
​
The Manhattan Project used the fissile element plutonium in its second line of research and development. Despite the fact that only trace amounts of plutonium may be found in nature, nuclear reactors are the most efficient means to produce substantial amounts of the material by bombarding uranium with neutrons. Uranium-238 undergoes transmutation into uranium-239, which quickly decays into plutonium-239 and eventually neptunium-239. Because only a tiny fraction of the uranium-238 will undergo transformation, the plutonium must be chemically separated from the rest of the uranium, any initial impurities, and the fission products.
​
​
​
​
The construction of a plutonium plant on a 500 m² site at Oak Ridge in March of 1943. It included the air-cooled X-10 Graphite Reactor, a chemical separation plant, and support facilities. The greatest difficulty was encountered with the uranium slugs produced by Mallinckrodt and Metal Hydrides. The Grasselli Chemical Company attempted to develop a hot dipping process, while Alcoa tried canning. The Metallurgical Laboratory eventually developed an improved welding technique with the help of General Electric, which was incorporated into the production process in October of 1943. The X-10 Graphite Reactor started to operate on the 4th of November, 1943, with about 27,000 kg of uranium, and by the end of the month the first 500 mg of plutonium were created. Modifications over time raised the power to 4,000 kW by July of 1944. X-10 operated as a production plant until January of 1945, when it was turned over to research activities.
​
​
​
​
​
​
​
​
​
​
​
​
​
​
​
​
​
​
​
​
​
​
​
Although an air-cooled design was chosen for the reactor at Oak Ridge to facilitate rapid construction, it was recognized that this would be impractical for the much larger production reactors. Initial designs by the Metallurgical Laboratory and DuPont used helium for cooling, before they determined that a water-cooled reactor would be simpler, cheaper and quicker to build. The design was not finished until the 4th of October, 1943; in the meantime, Matthias concentrated on improving the Hanford Site by building accommodations, improving the roads, building a railway switch line, and upgrading the electricity, water and telephone lines. However, steady progress was made and by June of 1944 production increased to the point where it appeared that enough canned slugs would be available to start the building of Reactor B on schedule in August of 1944. Watched by Compton, Matthias, DuPont`s Crawford Greenewalt, Leona Woods and Fermi, who inserted the first slug, the reactor was powered up beginning on the 13th of September, 1944. At first all appeared well but around 03:00 the power level started to drop and by 06:30 the reactor had shut down completely. The cooling water was investigated to see if there was a leak or contamination. The next day the reactor started up again, only to shut down once more.
​
Fermi identified the cause of the problem as neutron poisoning from xenon-135, which had a half-life of only 9.2 hours. Fermi calculated the nuclear cross section of xenon-135, which was 30,000 times that of uranium. DuPont engineer George Graves had deviated from the original design, but Fermi realized that by loading all 2,004 tubes, the reactor could reach the required power level and efficiently produce plutonium. Reactor D was eventually started on the 17th of December, 1944, and Reactor F on the 25th of February, 1945.
​
​
​
​
​
​
​
​
​
​
​
​
​
​
​
​
​
​
​
​
​
​
​
The chemists at the Metallurgical Laboratory in 1942 developed a lanthanum fluoride process (which is a type of ion selective electrode sensitive to the concentration of the fluoride ion) for separating uranium and plutonium, which was chosen for the pilot separation plant. A second separation process, the bismuth phosphate process, was developed by Seaborg and Stanly G. Thomson. Greenewalt favored the bismuth phosphate process (which was a process used to extract plutonium from irradiated uranium taken from nuclear reactors) due to the corrosive nature of lanthanum fluoride, and it was selected for the Hanford separation plants. After X-10 began producing plutonium, the pilot separation plant was put to the test and the efficiency was raised to 90%. At Hanford, top priority was given to the installations in the 300 area, which contained buildings for testing materials, preparing uranium, and assembling and calibrating instrumentation.
Work on the 300 area fell behind schedule due to the unique and complex nature of the facilities, and wartime shortages of labor and materials. Work began on 221-T and 221-U in January of 1944, with the former completed in September and the latter in December. The 224-T and 224-U buildings were completed on the 8th of October, 1944, and 224-B followed on the 10th of February, 1945. The purification methods that were eventually used in 231-W were still unknown when construction commenced on the 8th of April, 1944, but the plant was complete and the methods were selected by the end of the same year. On the 5th of February, 1945, Matthias hand-delivered the first shipment of 80 g of 95%-pure plutonium nitrate to a Los Alamos courier in Los Angeles.
​
Now, all that was needed was to create the actual bomb.
​
​
​
​
​
​
URANIUM


Image provided by Catalog.Archives.gov

GETTING THE DESIRED ORE
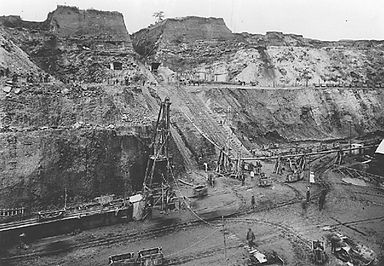
Shinkolobwe Uranium Mine
Image provided by NuclearMuseum.org
ISOTOPE SEPERATION
Oak Ridge hosted several uranium separation technologies. The Y-12 electromagnetic separation plant is in the upper right. The K-25 and K-27 gaseous diffusion plants are in the lower left, near the S-50 thermal diffusion plant. The X-10 was for plutonium production.
Image provided by Wikipedia.org
CENTRIFUGES
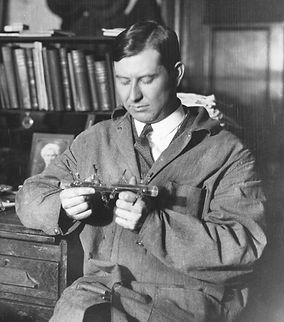
Image provided by Ans.org
ELECTROMAGNETIC SEPERATION
Calutron Girls were young women who monitored calutron control panels at Y-12. Gladys Owens, seated in the foreground, was unaware of what she had been involved in.
Image provided by Smithdray1.net
GASEOUS DIFFUSION
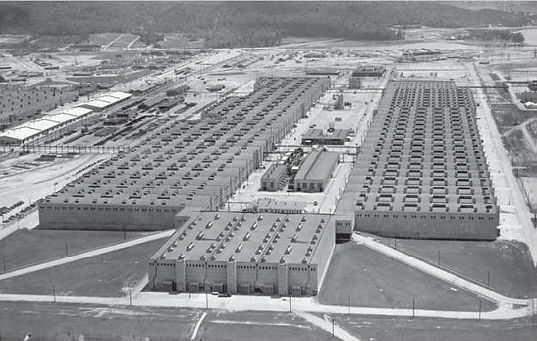.jpg)
Image provided by Wikipedia.org
THERMAL DIFFUSION
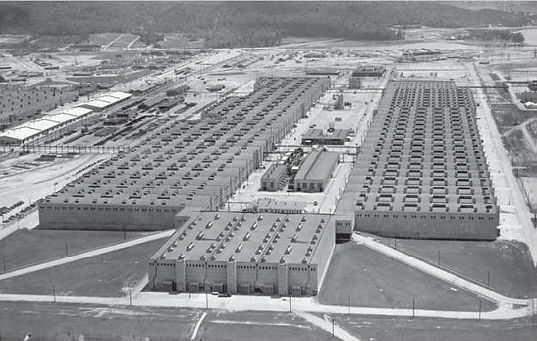.jpg)
Image provided by Nps.org
PLUTONIUM
X-10 GRAPHITE REACTOR
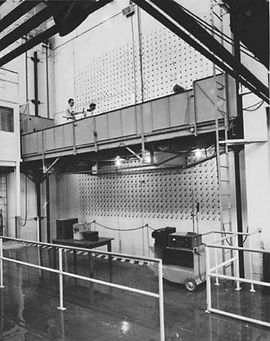
Image provided by Energy.gov
HANFORD REACTORS
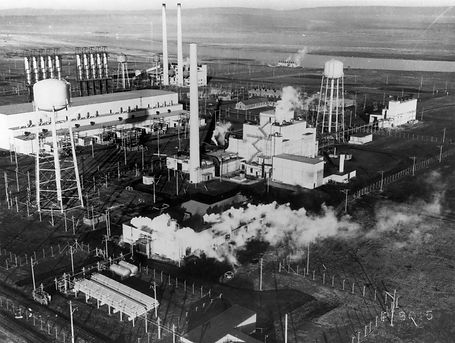